An insight to rocket propulsion
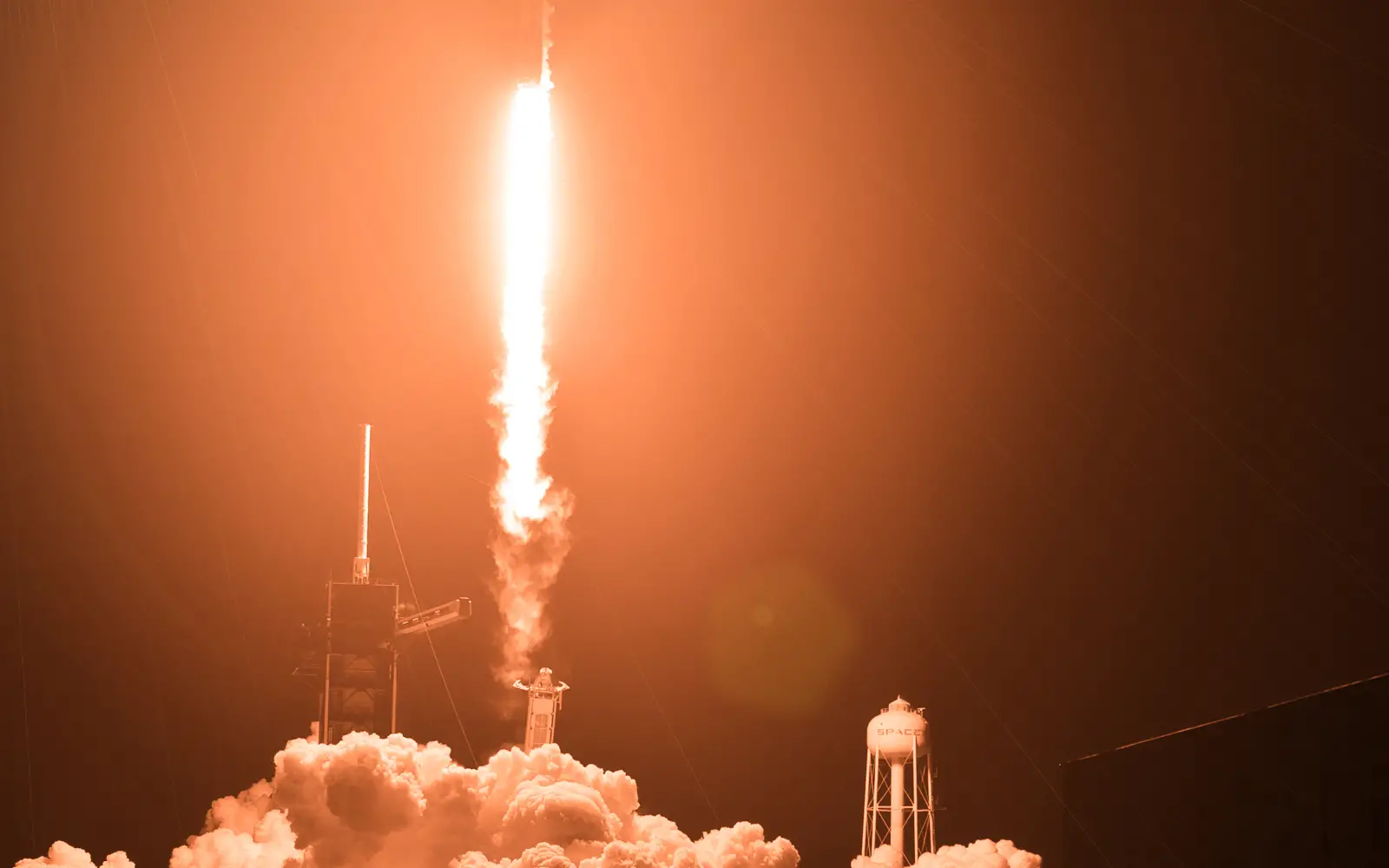
Konstantin Tsiolkovsky, born in Russia in 1857, was a rocket scientist who is known worldwide for pioneering the astronautic theory. This theory, as first proposed by Tsiolkovsky in 1898, involved the discovery of the concept to use rockets for space exploration. Tsiolkovsky went on to identify the potential to use a liquid propulsion method to allow launch vehicles to travel further into space. The speed the rocket would travel at, and the total distance (range) the rocket could achieve, would depend on the velocity of the exhaust gases released when the propellants burn to produce thrust.
Over the years this technology would continue to develop and still does so now. With the discovery of new technologies such as Skyrora’s Ecosene and access to innovative manufacturing processes such as DED 3D Printing and Carbon Composite Technology, we can develop and discover the increased potential of propulsion systems to allow efficient and more sustainable access to space.
How it works
Propulsion is the act of providing a force to move a body either from an initial point at rest or by changing the velocity of a body that is already moving. Rocket propulsion is a type of jet propulsion whereby thrust is produced upon the ejection of a propellant.
Propulsion systems are classified based on varying characteristics including the type of energy source used, the type and size of the vehicle, and the propellant choice. Most of the time this process involves the principle of thermodynamics with which a gas expands, causing the internal energy to be transformed into kinetic energy. The expanding gas applies pressure to the surrounding surfaces creating the downward force known as thrust.
A launch vehicle supplies us with a means to escape from Earth’s surface, but to do this a certain velocity is required to overcome the forces pushing the vehicle back towards the ground. Thrust, the propulsive force created by the ejection of matter at a high velocity, forces the rocket upwards. The velocity required by the propulsion system for lift-off is found by equating the kinetic energy of a moving body to the work required to overcome gravity. This velocity is often impractical for launching a rocket, as on ascent it is subject to many more extreme forces, so the propulsions systems implemented must be tailored to the changing velocity required to suit each stage of flight. This velocity will vary depending on the altitude the launch vehicle must reach, for instance, a vehicle headed for Low Earth Orbit (LEO) will have different requirements for its propulsion system to those heading into deep space for interplanetary missions. In the case of the Skyrora XL launch vehicle, the three-stage propulsion system can perform multiple sequential burns allowing us to service different altitudes, increasing our mission envelope.
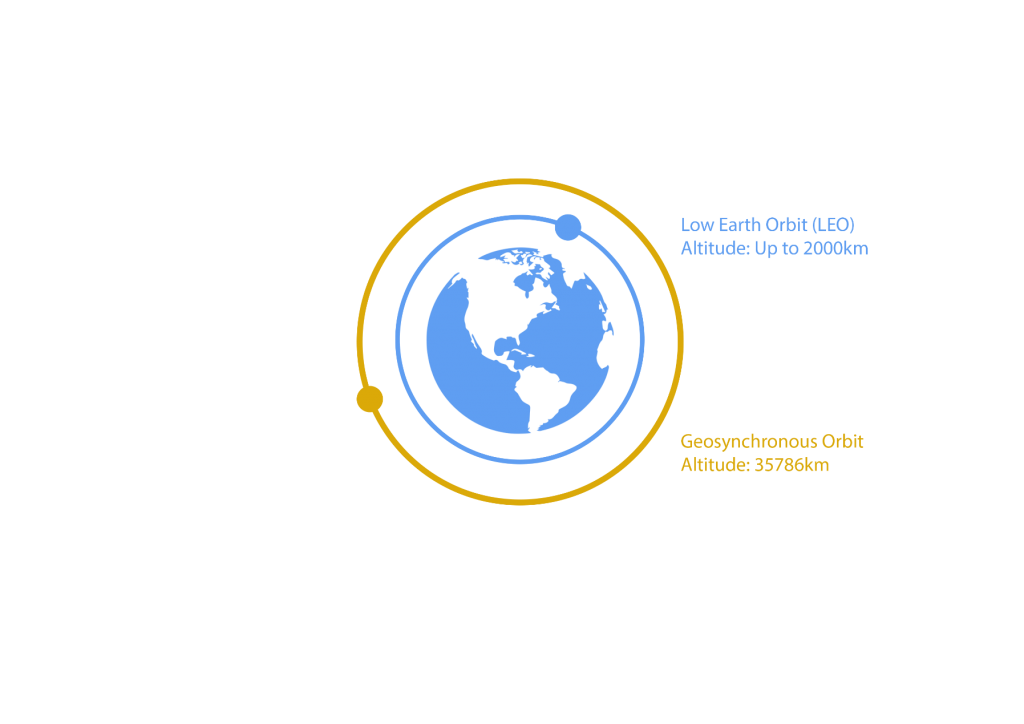
The principles involved
The concept of propulsion in space and rocket science stems from the understanding of mechanics, thermodynamics, and chemistry. Knowledge of these principles is essential in obtaining optimum results for the system responsible for launching a rocket into orbit. We rely on the total impulse during launch, which is calculated from the integral of the force produced by the thrust and the burning time of the propellant. Total impulse is proportional to the energy released by the propellant. Similarly, the specific impulse, which describes the efficiency of the rocket, is the value for total impulse divided by the weight of the propellant. The higher the specific impulse the more efficient the system, as more thrust is produced for the same amount of propellant.
There are two main types of propulsion system in space technology which have undergone extensive testing and experimentation since discovering the potential to use rockets for space exploration. The first of these is liquid propulsion, which uses a liquid oxidiser and a liquid fuel, combined in the engine’s combustion chamber and burned to produce a mostly clean-burning, non-toxic exhaust. This can produce a high maximum velocity for a fixed propellant mass. Alternatively, we can find solid propellants in use in many applications. Despite liquid propulsion being a preferred method, solid rocket fuels mixed with a solid oxidiser can allow for a simpler, more compact design which is ready to operate quickly.
The rocket engine
Rocket engines are reaction engines, where the mass of the fuel exerts a force in one direction and the rocket is in turn forced in the opposite direction due to the equal and opposite reaction force, as dictated by Newton’s third law of motion. The engine itself, considered here for liquid propellants, consists of a combustion chamber and nozzle. In the combustion chamber the fuel and oxidiser are combined to create the propellant mixture, and here they are burned to create a hot gas.
The hot gas passes through the nozzle, which is shaped such that the gas is compressed and then expanded, causing an increase in pressure and thus an increase in the velocity of the exhaust gas being forced downwards through the nozzle producing thrust. This shape of rocket engine nozzle is commonly known as a converging-diverging nozzle, where the exhaust velocity is increased from subsonic flow to supersonic flow.
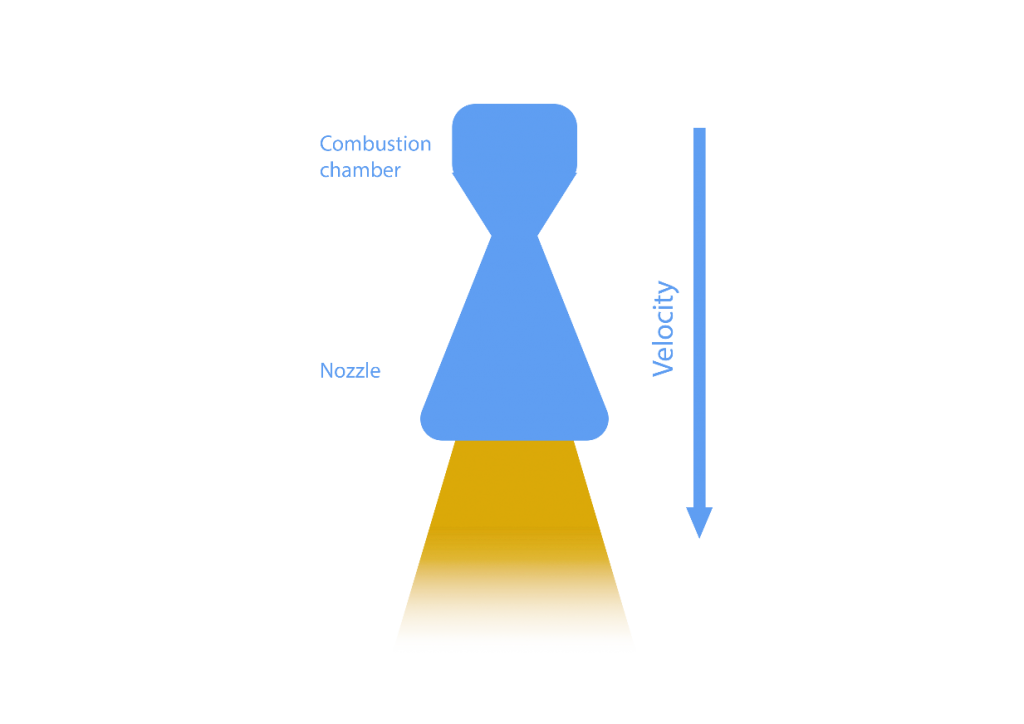
Each of the three stages in the Skyrora XL launch vehicle requires engines with varied specifications based on the system requirements. This is designed to place satellites into the exact sun-synchronous or polar orbit required. The first and second stages use a turbopump propellant feed system, whilst the third stage of Skyrora XL is pressure fed. The first stage uses 9x 70kN turbopump-fed engines. As the mission progresses to the second stage, Skyrora XL relies on the 1x 70kN Vacuum configuration engine. When the vehicle reaches higher altitudes the third stage, with its LEO engine and thrust of 3.5kN, is reignitable.
How do Skyrora do it?
The technology Skyrora are developing today follows in the footsteps of the Black Arrow programme, authorised in 1964, which saw the first-ever British satellite, Prospero, launched into orbit by a British rocket. The Black Arrow programme involved design processes to recycle as many technological components as possible from programmes previously developed, with the purpose of cost reduction and simplified development processes. It was a major milestone for the UK space industry. Find out more about Black Arrow here.
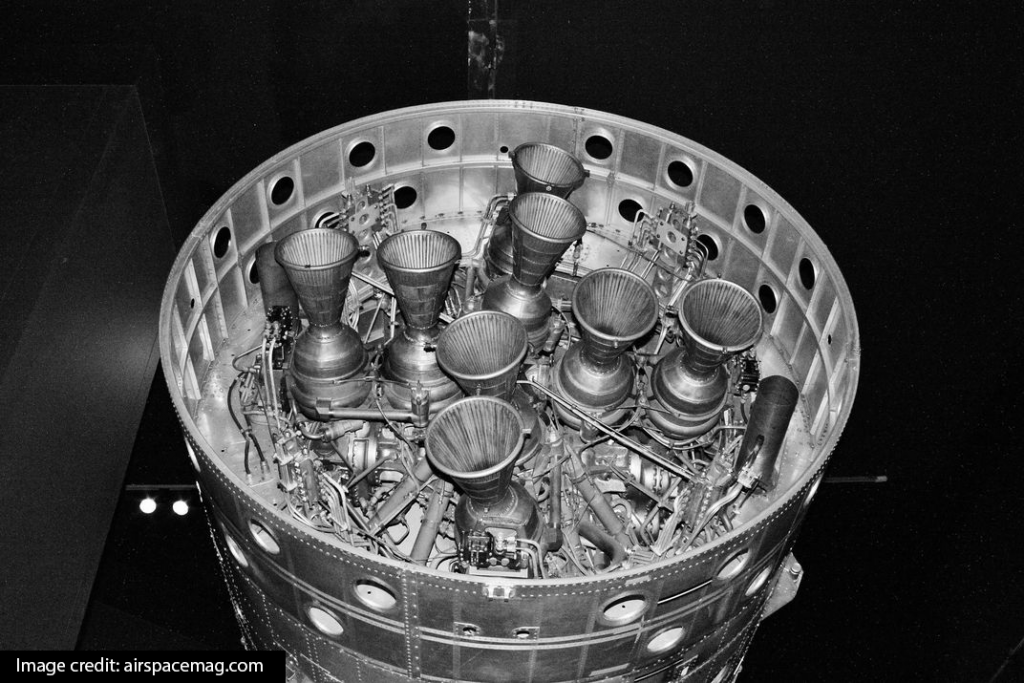
Having learned from this advanced technology, Skyrora’s current launch vehicles use a bi-propellant liquid fuel-oxidiser combination of HTP (high-test peroxide) and kerosene. The liquid propellant system allows more flexibility, namely being non-cryogenic meaning the propellant can be stored in the case of delayed launch and can launch with much less significant ground infrastructure compared to cryogenic systems. This is useful for Skyrora’s launches, particularly with unpredictable UK weather which oftentimes leaves a narrow launch window where the vehicle must be ready for a fuel-and-go system for launch. Skyrora’s Mobile Launch Complex also provides capabilities for a flexible, accessible launch service with decreased costs. Here all the necessary systems for launch are modular and can be quickly erected at the chosen launch site to make launch day procedures efficient, with no impact on the spaceport infrastructure, and leaves no footprint behind after launch.
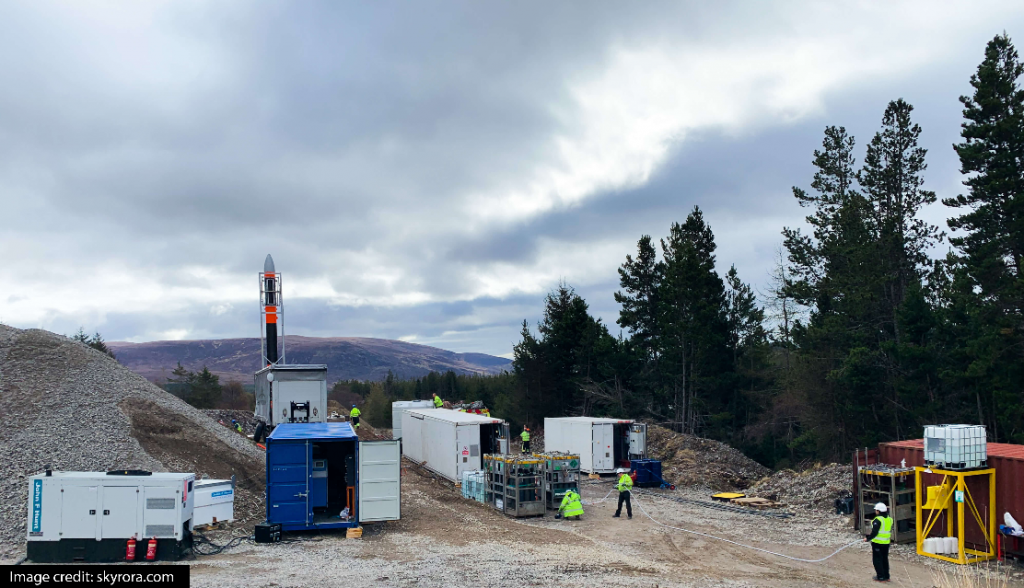
An environmental conscience
Keeping the ability to reduce Skyrora’s footprint in mind, Skyrora’s Modular Launch Complex is an important step forward in making the launch sustainable. This is just one of Skyora’s technologies that contribute to our goals for environmentally-friendly launch.
Compared to the burning of solid powdered metal fuels and other liquid fuel-oxidiser propellant ratios, Skyrora’s propellant mixture of hydrogen peroxide and kerosene is advantageous from an environmental perspective, using less kerosene to produce less CO2. On top of this, Skyrora continues to develop Ecosene, the replacement fuel made from non-recyclable plastics, which can reduce CO2 emissions by almost half during launch. Ecosene uses polypropylene (PP), polyester (PE), and polystyrene (PS) which are converted to this high-grade fuel found to be 1-3% more efficient than kerosene during testing. Find out more here.
References
1. George P. Sutton, Oscar Biblarz (2017). Rocket Propulsion Elements. Wiley.
2. NASA . (2021). Rocket Propulsion. Available: https://www.grc.nasa.gov/www/k-12/airplane/rocket.html. Last accessed 31st Dec 2021.
3. NASA. PROPELLANTS. Available: https://history.nasa.gov/conghand/propelnt.htm. Last accessed 8th Dec 2021.
4. National Space Society. Project: LEO. Available: https://www.eisacademy.org/course/view.php?id=81. Last accessed 50th Jan 2022.